The STORE&GO project
The need for energy storage
In 2015, 195 countries have signed the Paris climate agreement that aims at reducing global warming to 2 °C or in a best case scenario to 1.5 °C. To reach such targets, greenhouse gas emissions have to be reduced dramatically, as reflected in the EU’s ambitious climate targets. In order to meet these targets, energy production must come mainly from renewable sources. However, power supply from wind and solar will surpass demand at times – for instance, during sunny summer days – and vice versa. Energy has therefore to be stored to be made available when needed. This is where STORE&GO comes into play.
The project STORE&GO
Long-term and large-scale storage demands for high energy density, low costs and little self-discharging. One option is to use power-to-gas “PtG”, which allows for storing power by producing renewable hydrogen or renewable methane. Funded under Horizon 2020 from 2016 to 2020, STORE&GO was running three pilot plants with different innovative power-to-gas technologies. On top of the technology demonstration, the 27 European partners were investigating the potential of PtG in the European energy grid.
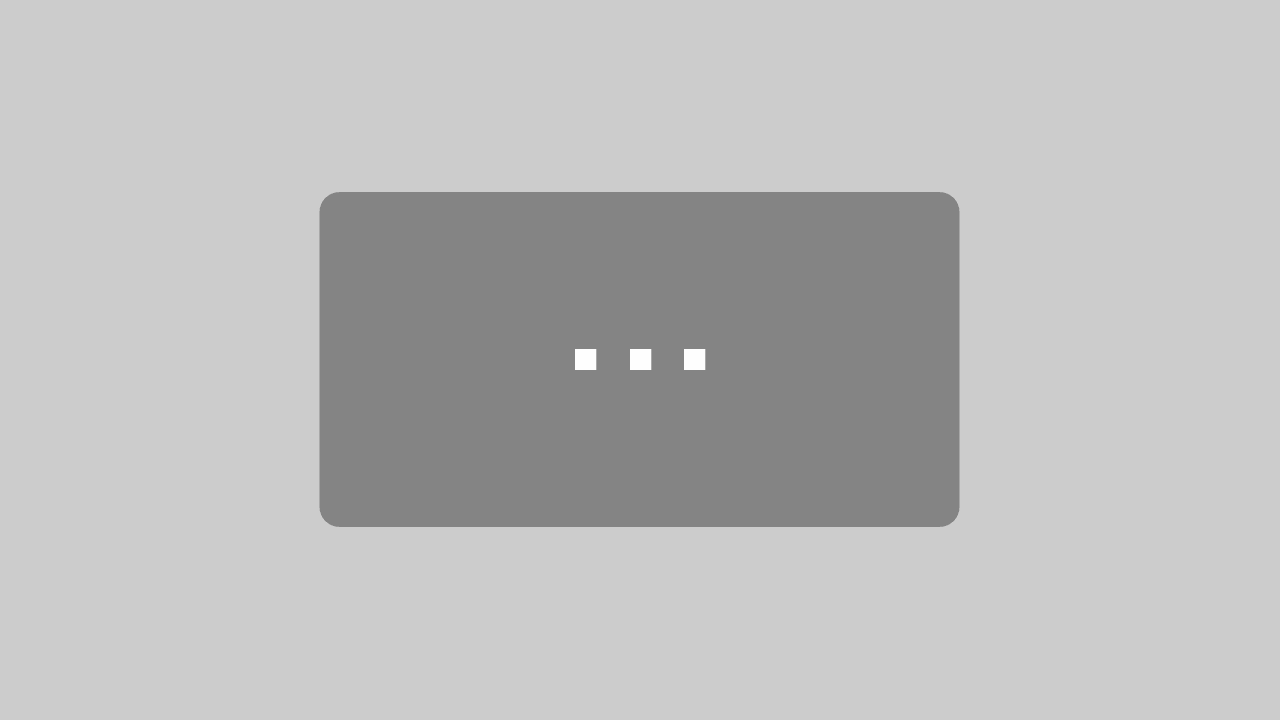
Mit dem Laden des Videos akzeptieren Sie die Datenschutzerklärung von Vimeo.
Mehr erfahren
Key Results of the STORE&GO project
In order to reach the EU’s 2050 climate targets, greening of electrons (power) so far seems on track. Greening of molecules (gases) however is lagging far behind, with only a few percentages of green-only and little perspective on serious progress towards 2030 targets and thereafter. This lack of green molecules is a serious shortcoming of the energy transition, since currently, energy molecules (gas/oil/coal) cover over 70% of the EU energy system. Instead of transporting fossil gas, the existing European gas grid is fit already today to transport green gases produced via power-to-gas – in the case of green methane even without technical adaptions.
The European energy picture in 2050 derived in the roadmap shows a wide range of 1860 TWh to 4700 TWh for the total annual gas demand, which needs to be covered predominantly by green gases. Our research did not show a low future gas demand, even with a high CO2 reduction target. Since even high electrification scenarios estimate 40–60% need of molecules in 2050, the 1860 TWh are assumed to be the absolute lowest estimation. Further investigations by the STORE&GO project conclude with higher gas demands. The top-down analysis finds a future gas demand of around 4400 TWh, and also the bottom-up analysis indicates it in the upper range of the estimated corridor.
Technology-wise, rapid development is ongoing in power-to-gas technologies. The main components of the PtG technology – electrolyser and methanation systems – show significant cost reduction potentials thanks to scaling effects and technological learning. Depending on the chosen electrolyser application, the specific investment costs for large scale systems could decrease respectively.
The consortium has identified three major categories of measures that need to be tackled in order to get power-to-gas to the level of market uptake. In addition to this, there are also several cross-cutting specific issues that should be addressed as soon as possible.
The evaluation concludes that power-to-gas currently finds itself in the valley of death, without a perspective of a functioning market for green gas on the other side of the valley. Several suggestions for fitting measures are given and categorised correspondingly. This can be done by creating specific support and subsidy schemes for both research and development as well as for investors, by granting feed-in remuneration for operators and by reforming regulation policies. Simultaneously, a market for green gases needs to be established, and here instruments such as a mandatory admixing quota with cross sectoral impact, penalizing CO2 emissions financially and providing a framework of guarantees of origin for renewable gases can be the options of choice. The latter is the internalisation of external costs and coordination of regulations in the electricity and gas markets, which the project partners see as mandatory for the energy transition. Such measures would result in economic incentives for investors and operators, which are missing today.
In a future perspective, the gas system will not only be confronted with one type of gas, and there are many politically driven strategic decisions influencing the future development. For example the question of import from outside or renewable gas production inside of the EU, CCS and CCU, to mention some. The decarbonisation of European energy can be considered as an opportunity to further strengthen European leadership on innovative energy technologies, energy-related transport technologies and services, as well as the application and implementation of matured and climate-friendly gas-related technologies. The results of this Roadmap underline this and show how power-to-gas could and should become a part of this future.
Detailed information and a realistic and effective strategy to assure a successful introduction of power-to-gas can be found in the deliverable report D8.10 as well as in the STORE&GO Roadmap Brochure.
In STORE&GO the externalities of power-to-gas were investigated. It was shown that PtG has various positive externalities (including environmental, health, grid cost and balancing and energy security benefits) that provide benefits to other stakeholder groups (like network operators and the broader society) in the market system, but not directly result in a commercially viable business case for PtG investments. It was assessed that these positive externalities could serve as a rationale for policy change and could be monetised and redistributed to cover the higher PtG investment costs and risks, in order to further develop PtG in the EU.
Further detailed information on externalities of PtG and different policy pathways for PtG in the EU can be found in deliverable report D8.8.
The STORE&GO project indentified suitable locations for power-to-methane in Europe by coupling CO2 sources (biogas and industrial plants) with locally available renewable energy (RE) sources (wind and utility-scale PV). By using publicly available data on renewable energy generation and biogas production sites a highly resolved geo-information data model was generated and used to calculate the local correlations of CO2 availability and renewable energy production throughout Europe. CO2 sources exhibiting large-scale renewable energy generation within a radius of 10 km were identified as potential PtM energy conversion sites, enabling local energy sector coupling and the production of methane. Additional information such as the locations and distances between potential energy conversion sites and substations from the electricity system or other infrastructures can be visualised and included in the assessments. The local land use e.g. in terms of the exact polygons of agricultural land may be utilised for example to model future erections of photovoltaic plants, as presented in several case studies.
Furthermore, projections of methane production potential up to 2050 were carried out on national level. Since the design of this study centres the development of power-to-methane plants around existing CO2 sources, the production potentials were assessed on the basis of two scenarios for the future availability of CO2: alimited CO2 availability scenarioand a balanced CO2 availability scenario, respectively.The resulting power-to-methane maps provide insights into the possibilities of developing a methanation technology rollout starting from the status quo of renewable energy infrastructure in Europe.
Further detailed information on the assessment of suitable locations for power-to-methane and the projections of methane production potential up to 2050 can be found in deliverable report D8.9.
Experience from STORE&GO has shown that PtG is technically feasible and reasonable. The extent to which the market launch and further development of the technology progress depends on the profitability and above all on the investment costs of the plants. For this reason, a sub-project of STORE&GO was devoted to techno-economic analysis. In addition to the key technological features – such as the current state of the art and future projects – new developments, technologies and materials as well as possible future areas of application were analysed.
Our analysis show that there is considerable potential for reducing costs, especially for electrolysers. This is based on scaling effects and learning curves. Models show that investment costs for all technologies will fall sharply in the future (Figures below).
Until PtG establishes itself as a technology on the market, so-called learning investments will be necessary. Among the currently available technologies, the Proton Exchange Membrane Electrolysis Cell (PEMEC) has the lowest investment costs. The greatest effects from learning and scaling effects are expected for solid oxide electrolysis cells (SOEC) in the future.
For catalytic and biological methanation, a similar cost degression through learning and scaling effects is expected. According to the STORE&GO experts, the two methods will differ only marginally in terms of their cost reduction potential over time (see figure below).
For further information: deliverable report D7.5
The STORE&GO project developed and designed the training programme “Power to (the) Molecules – from technology to market uptake”. Both an online and a classroom programme are part of the 3-day programme, which includes the technical, economic, regulatory, environmental and social aspects of the energy conversion/storage technology Power-to-Gas. Over the course of the project eight editions of the programme were successfully completed. As the project STORE&GO, the programme is set up to put the technology into the wider perspective of the energy system, and to analyse what constitutes its business case and long-term perspective in contributing a future sustainable and reliable energy system in Europe. The integration of energy systems (electric, molecules and heat) requires a multilateral understanding between the various stakeholders involved. By sharing knowledge about these various aspects the STORE&GO project aimed to contribute to a more effective and cost-efficient energy transition.
The main message of the training was:
- Sector coupling is necessary to transform the EU into a carbon-neutral economy by 2050
- PtG is a mature technology enabling closer integration between power and end-use sectors
- PtG is not the only pathway to achieve carbon neutrality
- Any changes to the existing regulatory framework should be technology-neutral and should enable the market participants and investors to decide which technology could bring the greatest value for the end-users at the lowest cost.
The training programme is the subject of the deliverable reports D9.4 and D9.7, where further detailed information is available. A public training programme is available for download here.
One of the specific issues that have been analysed within STORE&GO is the expected carbon footprint of synthetic methane (SNG) from the project demo sites. Xun Liao and Victor Godina, both members of Prof. François Maréchal’s research group at the EPFL (École Polytechnique Fédérale de Lausanne), calculated the life cycle carbon footprint of SNG from the STORE&GO demo plants and compared it with the footprint of fossil gas. The calculations take into account the whole PtG process: from plant construction and operation to combustion of the product.
The study shows that the carbon footprint of SNG from PtG has high variability depending on geographical location, system configurations, electricity generation mix and CO2 sourcing. Other influencing factors are opportunities for heat integration and surplus heat valorisation, maturity and efficiency of the technology, and scale of production. For the specific case that the small-scale demo plant in Troia is powered by the regular Italian electricity mix – which includes high-carbon energy sources – the production and combustion of one cubic meter of such SNG generates CO2 emissions of 14.1 kgCO2eq/m3 (blue bar in the graph). If, however, electricity for the Troia plant comes exclusively from the nearby wind and solar installations, the carbon footprint is reduced by 85% to a low number of 2 kgCO2eq/m3 (light green bar). Future large-scale PtG plants will benefit from further optimization measures, such as economies of scale, heat valorisation, improved materials sourcing and improved efficiency of the electrolysis. With these measures, the carbon footprint of future SNG can be reduced even further to only 0.4 kgCO2eq/m3 (dark green bar). For comparison, the production and combustion of one cubic meter of fossil natural gas emits six times more greenhouse gases.
As one of the scientists involved in STORE&GO, Herib Blanco from the Center for Energy and Environmental Sciences at the University of Groningen modelled the
European energy system in the year 2050 using a tool developed at the Joint Research Centre based on cost optimization. Given the large uncertainty on how the future can unfold, an extensive sensitivity analysis was done to cover the wide range of possibilities. The objective was to identify the role PtG can play in the future and to identify the drivers and barriers for the technology. Modelling parameters included CO2 emission target, availability of carbon capture and storage, biomass potential and technology performance, among others.
21 out of the 55 low carbon scenarios show that a synthetic methane capacity within a range from 40 to 200 GW might fit in the future energy systems, which corresponds to 5% and 30% of the future overall gas demand, respectively. This means that there is a multitude of scenarios where PtG can play a crucial role. Therefore, research and development has to be continued so that the technology will be available in coming years to support the transition of the energy system to a world free of fossil carbon.
Among the model parameters that showed a strong impact on the deployment of PtG, these are the main drivers:
- Ambitious (+95%) CO2 reduction targets
- Limited availability of CO2 underground storage
- Limited biomass potential
- High process efficiency and low technology cost
- Use of liquefied methane for navigation and heavy-duty transport
- Costly, difficult or heavily delayed expansion of the electricity grid
Detailed information on the scenarios, models and results can be found in deliverable report D6.3.
STORE&GO investigated how an interaction between the future electricity transmission system and the power-to-gas technology could be shaped. By considering two out of the three scenarios forecast by ENTSO-E for the year 2040, the obtained impact of PtG on the network is not negligible and in all cases allows to improve the value of RES dispatched. The amount of dispatched RES was compared between two cases: (i) all network investments implemented, and (ii) 20% of the network investments redirected to PtG.
The results show that the investment in PtG allows an increase of the amount of RES dispatched at the European level. The increase lies in the range between 9 % and 20 %, depending on the month and the scenario considered. The presence of PtG in the European transmission system is beneficial also for its economic operation: in fact, the average electricity cost is decreasing between about 13 % and 23 % thanks to the increase of the share of RES injected into the system.
The detailed information on how the integration of power-to-gas in the future electricity transmission system was developed can be found in deliverable report D6.6.
An interview with Gijs Kreeft, Faculty of Law, University of Groningen Gijs Kreeft is a researcher within STORE&GO and staff of the Groningen Centre of Energy Law. His research focuses on legal and regulatory developments regarding Power-to-Gas, systems integration, and energy storage. One of the main objectives of the STORE&GO project is to identify legal and regulatory challenges for the deployment of power-to-gas and production of synthetic methane, both at the level of the European Union (EU) as well as at a national level for the host countries of the STORE&GO pilot plants. Studies also focus on legal measures facilitating the injection of synthetic, or substitute, natural gas (SNG) into the gas network. We asked Gijs Kreeft from the RUG University of Groningen about his work, and the challenges and possible solutions he has identified so far.
What are the challenges when it comes to licensing and regulatory regimes?
Kreeft: PtG is associated with various new concepts which are not yet (sufficiently) considered under EU and national legislation. For example, energy storage as an asset, and hydrogen and SNG as renewable energy carriers, have only recently gained attention from legislators. These new technical developments lead to various fundamental legal issues which need to be resolved. For instance, the question whether network system operators or gas storage system operators are allowed to run a PtG facility. Or whether authorisation procedures should consider PtG plants as chemicals-producing installations rather than installations that produce energy commodities. It is also necessary for legislators to define if, and under which circumstances, PtG is a gas producing activity instead of an energy or gas storage technology. Finally, due to the often fragmented and sectoral nature of energy legislation, the technical trend towards the coupling of energy sectors is not reflected in the legal framework.
Why do you think is there so much left open?
Kreeft: PtG is a relatively new technology and is still in the development phase. As is often the case: law follows innovation. Another issue is awareness. As policy-makers do not yet fully comprehend the PtG process and its potential for the transition towards a low-carbon energy system, they tend to adopt a ‘wait and see’ attitude. Consequently, PtG and SNG are not sufficiently considered in renewable energy promotion policies. We observed, for example, that electricity and heat from SNG is generally not considered as ‘renewable’. At a more structural level, policy-makers are still struggling with the question how to integrate technologies which connect energy systems through conversion. This leads to the current situation that in some cases, PtG plant operators and the actual final consumers both pay network tariffs and surcharges, leading to double taxation.
What can politics and lawmakers do to improve the framework?
Kreeft: Most important, we need a structural and fundamental shift in the political mind-set, through which we start to see the energy system not as being fragmented between electricity, gas, and heat, but instead as one single integrated system. The legal field is just starting to adapt to this new way of thinking. At the ‘issue-level’, we identified various issues. Among which are the necessity to develop harmonised rules on guarantees of origin, which take into account the need for seasonal storage, an evaluation of permit procedures, an end to double-taxation practices, and updated support schemes. Another efficient measure could be the introduction of a carbon tax, which should make renewable gases more competitive. Last but not least, R&D has to be further supported to strengthen the advantages of PtG further.
The legislative and regulatory framework of power-to-gas in Germany, Italy and Switzerland is discussed in detail in deliverable reports D7.2 and D7.3.
In STORE&GO also social factors were investigated such as the social acceptance of the power-to-gas technology. Therefore surveys were conducted in Austria, Germany, Italy and Switzerland. These surveys included a choice experiment examining the preferences of European households with respect to PtG and alternative energy infrastructures. Further on, the survey also collected information on household knowledge and attitude with respect to renewable energy, as well as their current socio-demographic characteristics and their experiences with respect to their electricity provider and consumption issues (power outages, delays in bill payment, etc.).
Results from the analysis show that solar farms and power-to-gas infrastructure increase acceptance of local energy communities, while wind farms have an ambiguous effect, and introduction of gas power plants and power lines decreases acceptance. Additionally, we investigated whether stated support from political opinion leaders at the local, national, and EU levels can increase the acceptance of renewable energy systems. Results suggest that Italian choices can be influenced by the opinions of EU and national governmental bodies, and that Swiss choices are sensitive to the opinions of local politicians.
The social and public acceptance determinants are discussed in detail in deliverable report D7.8.
STORE&GO assessed the economic aspects of SNG injection for different combinations of SNG plants and gas networks, as well as of optimised operation schemes for the gas networks. Next to the demo plants and the connected gas grids from within this project, combinations of several typical test grid topologies and increased plant sizes are considered, when calculating costs for injecting SNG under different network operation schemes. In addition, the capacities of the gas infrastructure and the connected underground storages for long term storage of currently natural gas, in the future SNG, are shown.
The energy demand and its associated costs for injecting SNG into the gas infrastructure depend mainly on the demand of compression. The main influencing parameters for the compression are the ratio of the operating pressure (OP) of the power-to-gas (PtG) plant to the OP of the respective gas grid as well as the considered volume flow. Therefore the connection of a PtG plant with a low outlet pressure to a high pressure gas grid causes the highest energy demand and costs, whereas the connection to a low pressure distribution grid causes no or reduced demand for compression.
To optimize the injection of SNG into the gas grid, besides the selection of suitable plant concepts for the respective gas grid, the network operation schemes can be adjusted to whether increase the capacity for injecting renewable gases or to lower the energy demand for compression. The energy demand for gas compression can be reduced by lowering the OP of the grids, the installable PtG system output can be increased using the dynamic storage capacity of the grid. Both possibilities for adjustment therefore have an opposite effect on the costs for compression.
The investment costs for the injection plant depend mainly on the required compressor system, which is depending mainly on the inlet and outlet pressures, the volume flow to be compressed and the resulting drive power. Therefore, a selection of suitable plant concepts for the respective gas grid could also decrease the costs for the overall system.
The biggest advantages, when injecting SNG into the gas grid, are the opportunity to defossilize the gas sector, and the access to a huge storage capacity for especially medium and long-term storage. The storage capacity of the gas grid itself is called linepack and depends mainly on the minimum and maximum pressure of the gas grid. This can be used in particular to optimize the grid operation for larger capacities for SNG or for reduced effort for injection of SNG. For long term storage the gas grid grants access to the gas underground storages. With a planned overall gas storage capacity of about 1 300 TWh in the year 2025, a share of about 25 % of the current yearly gas consumption of EU-28 can be stored – and therefore also large quantities of SNG.
Detailed information on the economic analysis of SNG injection can be found in deliverable report D5.10.